2Department of Pharmacology, The Second People’s Hospital of Lianyungang, Jiangsu, China
3Department of Internal and Pediatrics, School of Clinical Medicine, Qilu Medical University, Zibo, Shandong, China
Abstract
Background: The current study aims to identify the key pathways and potential therapeutic targets for pulmonary arterial hypertension (PAH) and to further evaluate the anti-PAH effects of isorhamnetin.
Methods: The dataset of gene expression profiling for PAH (GSE113439) was downloaded from the gene expression omnibus (GEO) database. Isorhamnetin target genes were extracted from the comparative toxicogenomics database (CTD). Various bioinformatics methods were employed to identify the core pathways associated with PAH and potential intervention targets. Molecular docking was conducted between the interacting target and the candidate compound, isorhamnetin.
Results: One thousand nine hundred sixty-two upregulated genes and 642 downregulated genes were identified. Molecular complex detection analyses revealed that the significant biological processes associated with upregulated genes included DNA damage response, mitotic cell cycle, and chromosome organization. In contrast, the significant biological processes related to downregulated genes encompassed cellular response to growth factor stimulus, response to growth factor, and blood vessel development. Immune infiltration analysis indicated that PAH is associated with significant changes in the distribution of immune cells and differential expression of immune checkpoints. Furthermore, 58 isorhamnetin targets were extracted from the CTD, and we identified 1 interacting gene, NFE2L2, among the differentially expressed genes (DEGs), DEGs related to ferroptosis, and isorhamnetin targets. Isorhamnetin demonstrated strong affinities with vascular endothelial growth factor (VEGF) receptors and transcription factors (ATM and ZNF24) associated with VEGFs, as well as the ferroptosis protein NFE2L2.
Conclusions: Pulmonary arterial hypertension is characterized by a series of abnormalities in downstream molecular signaling pathways, including DNA damage, immune dysregulation, VEGF signaling deficiency, and the ferroptosis process. These may represent the core pathophysiological mechanisms of PAH. Ferroptosis-related genes, such as NFE2L2 and TF (ATM, ZNF24) associated with VEGFs, are potential therapeutic targets that contribute to the mechanisms mentioned above. Isorhamnetin is a promising candidate compound for the treatment of PAH.
Graphical Abstract
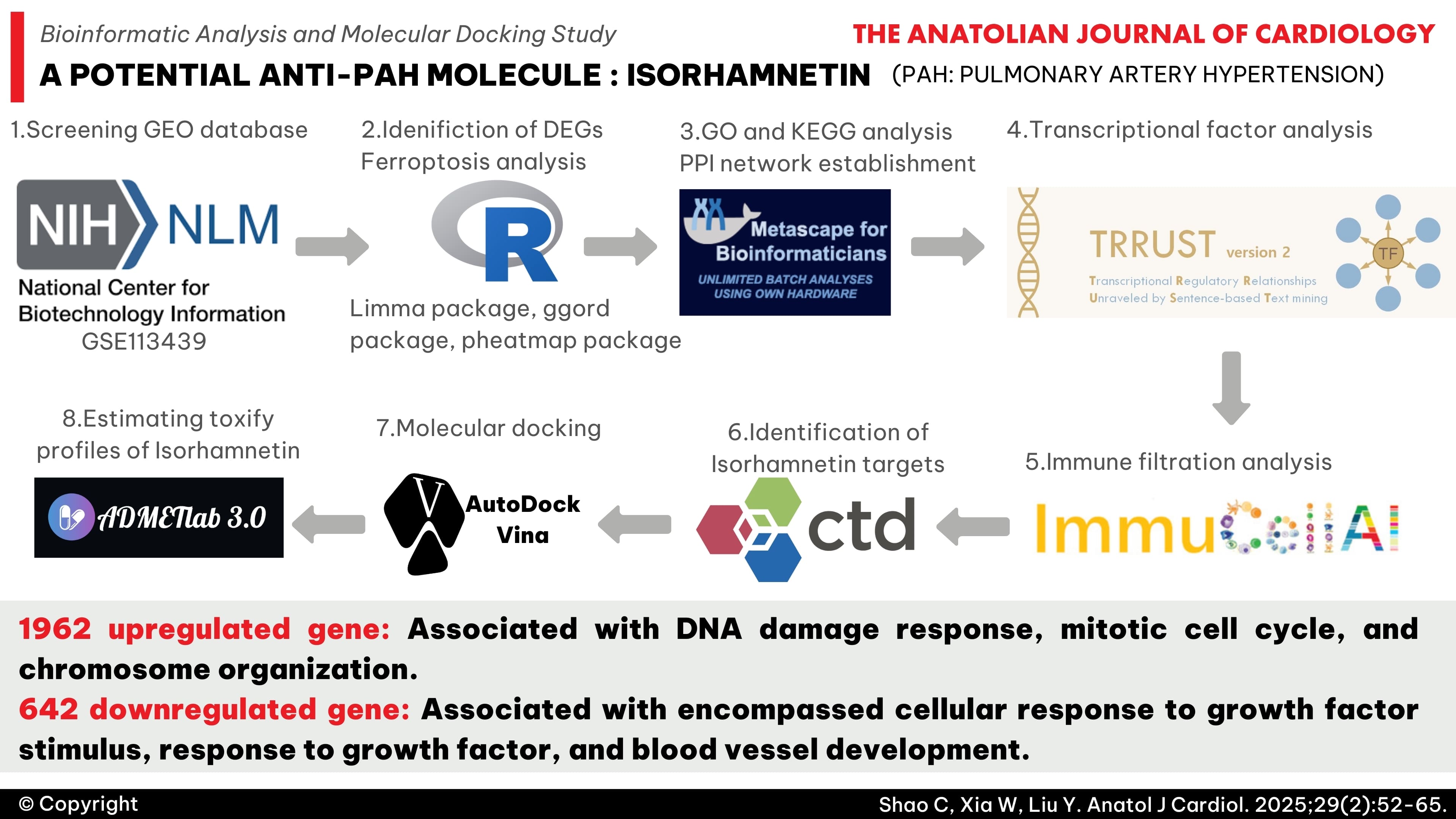
Highlights
- Based on network pharmacology, bioinformatics analysis, and molecular docking studies, we found that pulmonary artery hypertension (PAH) is associated with a series of abnormalities in downstream molecular signaling pathways, including DNA damage, immune dysregulation, vascular endothelial growth factor (VEGF) signal deficiency, and iron-induced apoptosis. Isorhamnetin emerges as a promising candidate compound for the treatment of PAH in these aspects.
Introduction
Pulmonary artery hypertension (PAH) is a severe, progressive disease that results in ongoing right ventricular remodeling and right heart failure. Pulmonary artery hypertension is characterized by increased pulmonary vascular resistance (PVR), arterial remodeling,
The mechanisms of PAH have been extensively studied in recent years; however, therapeutic targets and preventive methods have not yet been fully identified. Ferroptosis is a newly discovered form of cell death that differs from necrosis, apoptosis, and autophagy.3 It is primarily induced by iron-dependent lipid peroxidation, which leads to mitochondrial contraction, rupture of the mitochondrial membrane, cellular respiratory dysfunction, energy metabolism deficiency, and DNA damage.4,
In recent years, approximately 4000 plant-derived flavonoid compounds have been identified, many of which possess a variety of medicinal properties.9 Isorhamnoin is a specific class of flavonoid compounds primarily extracted from the fruits of “
Methods
Dataset
We conducted a gene expression profiling analysis of pulmonary arterial hypertension (GSE113439) using the gene expression omnibus (GEO) database (
Differentially Expressed Genes
All microarray data were downloaded from the GEO database (
Ferroptosis
Microarray data were downloaded from the GEO database (
Functional Enrichment and Protein-Protein Interaction Network
Gene Ontology (GO) is a widely utilized tool for annotating genes with their functions, particularly in the areas of molecular function (MF), biological processes (BP), and cellular components (CC). The Kyoto encyclopedia of genes and genomes (KEGG) enrichment analysis serves as a valuable practical resource for investigating gene functions and associated high-level genomic information. To gain a deeper understanding of the functions of differentially expressed genes (DEGs), functional enrichment analysis and a protein-protein interaction (PPI) network for the DEGs were established using Metascape (version: v3.5.20240101). Metascape is a web-based portal designed to provide comprehensive gene list annotation and analysis resources for experimental biologists. It is an effective and efficient tool for researchers to analyze and interpret OMICs-based studies in the era of big data. This online tool includes modules such as enrichment clustering, protein network analysis, multi-gene list meta-analysis, and transcription factor analysis. Additionally, the cytoscape plug-in for molecular complex detection (MCODE) was employed to explore key functional modules within the PPI network.19
Transcription Factor Analysis
Transcription regulatory relationships unraveled sentence-based text mining (TRRUST) (
Immune Filtration Analysis
ImmuCellAI (Immune Cell Abundance Identifier) is a tool designed to estimate the abundance of 24 immune cell types from gene expression datasets, including RNA-Seq and microarray data. These 24 immune cells comprise 18 T-cell subtypes and 6 additional immune cells: B cells, NK cells, monocyte cells, macrophage cells, neutrophil cells, and dendritic cells (DCs).21,
In our study, we analyzed the immune infiltration of PAH using a specialized tool. Additionally, we compared the expression levels of 10 immune checkpoint genes between PAH samples and control samples. Microarray data were downloaded from theGEO database (
Isorhamnetin Targets
Comparative toxicogenomics database (CTD) is a comprehensive, publicly accessible database designed to enhance our understanding of how environmental exposures affect human health. It offers meticulously curated information on chemical-gene/protein interactions, chemical-disease relationships, and gene-disease associations. This data is integrated with functional and pathway information to support the development of hypotheses regarding the mechanisms underlying diseases influenced by environmental factors. In this study, we utilized CTD to identify the targets of Isorhamnetin.26
Molecular Docking
NFE2L2 was identified as a common gene among DEGs, ferroptosis, and isorhamnetin, those interacting with isorhamnetin. Consequently, we conducted molecular docking to assess the binding affinities between isorhamnetin and NFE2L2. The crystal structures of the Nrf2/NFE2L2, VEGFR, ZNF24, and ATM proteins used for docking were obtained from the protein data bank (PDB), which is accessible at
The 3-dimensional (3D) structure of the small molecule isorhamnetin was downloaded from the PubChem database (PubChem CID: 5281654), and energy minimization was performed using the MMFF94 force field. AutoDock Vina 1.2.3 software, developed by the Scripps Research Institute, was utilized for molecular docking. Prior to docking, PyMol 2.5.5 was employed to remove water molecules, salt ions, and small molecules.31 The coordinates for docking are listed in
Estimating Toxify Profiles of Isorhamnetin
ADMETlab 3.0 (available at:
Statement
We do not use artificial intelligence (AI) assistive technologies (such as large language models (LLMs), chatbots, or image creators).
The study is secondary and has no ethical implications.
Results
Identification of DEGs in PAH
Enrichment ofDEGs and establishment ofPPI network
To investigate the biological functions of these DEGs,GO andKEGG pathway enrichment analyses were conducted on 1962 upregulated genes and 642 downregulated genes associated with PAH, utilizing the Metascape online tool. The results revealed that the top functional GO terms for upregulated DEGs included the mitotic cell cycle and DNA damage response, while the downregulated DEGs were primarily associated with vasculature development and cellular responses to growth factor stimuli (
Differentially Expressed Genes of Ferroptosis-Related Genes
Twenty-four ferroptosis-related genes were identified and analyzed. The results indicate that genes associated with ferroptosis, such as
Transcription Factors
The results of the KEGG analysis and MCODE estimation indicated that the cellular response to growth factor stimulus, response to growth factor, and blood vessel development were the top pathways affected in the down-regulated genes associated with PAH. It is well established that VEGF signaling plays a crucial role in blood vessel activities. These findings suggest a potential reduction in VEGF signaling. Consequently, we investigated theTFs regulating the expression of VEGF-A, VEGF-B, and VEGF-C by consulting the TRRUSS database. We identified 58 TFs for VEGF-A, 7 TFs for VEGF-B, and 2 TFs for VEGF-C, as presented in
Immune Characteristics in Pulmonary Arterial Hypertension
The raw microarray data of PAH were input into the immune cell abundance identifier. The results indicated that the distribution and infiltration of immune cells in PAH samples were altered compared to control samples. The affected immune cells included dendritic cells (DC), macrophages, natural killer (NK) cells, neutrophils, CD8+ T cells, inflammatory Helper T 17 cells, T follicular helper (Tfh) cells, cytotoxic T cells, central memory T cells, and effector memory T cells. The infiltration score slightly increased in PAH samples (0.866) compared to control samples (0.837) (
Identification of Isorhamnetin
We extracted 58 isorhamnetin-interacting genes from the CTD. These genes are highly enriched in various biological processes, including responses to hormones, response to xenobiotic stimuli, response to oxidative stress, inorganic substances, peptides, and chemical stress. Additionally, they are involved in the regulation of inflammatory responses, responses to molecules of bacterial origin, toxic substances, and lipopolysaccharides (
Binding Capacity of Isorhamnetin to NFE2L2, VEGFR, ATM, and ZNF24
Molecular docking simulation technology is a convenient and effective method for exploring the interactions between small molecules and target proteins. Therefore, we utilized Vina 1.2.3 software to estimate the binding affinity of isorhamnetin to NFE2L2, VEGFR, ZNF24, and ATM. The results of the molecular docking indicate that chemical-protein interactions with a docking energy of less than 5 kcal/mol suggest a strong binding affinity.34
Isorhamnetin forms hydrogen-bonding interactions with NFE2L2 at specific amino acid sites, including PHE-490, ARG-499, ASN-482, and MET-485. The establishment of these hydrogen bonds enhances the binding affinity between proteins and small molecules. Additionally, Isorhamnetin exhibits hydrophobic interactions with NFE2L2 at the amino acid site MET-485, which may contribute to strong van der Waals forces between the molecules. The calculated affinity score of −6.869 kcal/mol between isorhamnetin and NFE2L2 indicates a favorable binding capacity of Isorhamnetin to NFE2L2 (
Toxicity Prediction of Isorhamnetin
We conducted ADMET predictions using ADMETlab 3.0 and found no acute toxicity associated with the oral administration of isorhamnetin. While there is no validated evidence of carcinogenicity, skin sensitization was identified (see
Discussion
Pulmonary arterial hypertension, a well-studied form of pulmonary hypertension, is classified as pre-capillary pulmonary hypertension. Advances in genetics and molecular medicine have led to the identification of genetic variations and susceptibilities associated with the onset of PAH. Factors such as genetic mutagenesis, DNA damage and repair, and the activation of cell death pathways contribute to the pathological processes underlying PAH. The most extensively studied mutated gene is BMPR2. In Western populations, 70% to 80% of patients with hereditary PAH and 10% to 20% of patients with idiopathic PAH (IPAH) have been found to carry mutations in the BMPR2 gene. These mutations are associated with early onset, severe clinical phenotypes, and poor outcomes. Although the exact causes and mechanisms of PAH remain unclear, it is generally accepted that multiple factors, pathways, and processes are involved. Currently, molecularly targeted therapies such as ambrisentan and tadalafil are employed to improve clinical conditions or exercise tolerance. However, there are still no effective drugs available to delay disease progression. Therapeutic interventions for PAH primarily focus on managing pathophysiological processes and controlling complications associated with the condition. Therefore, identifying key regulatory genes and functional pathways is essential.
In this study, we utilized GSE113439 to analyze the DEGs associated with PAH. We identified highly expressed genes in PAH samples that were significantly enriched in biological pathways related to DNA damage response, the mitotic cell cycle, and chromosome organization. Additionally, we observed notable changes in the expression levels of genes associated with ferroptosis. These findings suggest an activation of cell death pathways, cell protection mechanisms, and cell cycle progression in the development of PAH. Conversely, downregulated genes were significantly enriched in pathways associated with cellular responses to growth factor stimuli, responses to growth factors, and blood vessel development. It is well known that pulmonary artery endothelial cell (PAEC) dysfunction is the most common inducer of the occurrence and progression of PAH, and that VEGF signaling contributes to these functional processes.35 Chakraborty et al35 discovered that the loss of microvessels and the reduction ofVEGF-induced tip cell formation were crucial mechanisms in PAH.36 We interpreted the enrichment results of downregulated genes as indicating a decrease in vascular VEGF signaling. Consequently, we conducted a search forTFs that regulate VEGF-A, B, and C, identifying 7 common TFs with validated functions that overlapped with DEGs. Among these common TFs, ATM and ZNF24 are involved in repressing VEGF-A transcription. These 2 genes could potentially serve as targets for modulating reduced VEGF signaling.
Recent studies have confirmed that patients with PAH exhibit iron metabolic abnormalities, which are correlated with a decline in athletic performance, decreased survival rates, and the worsening of clinical symptoms.5,
Isorhamnetin (3-methylquercetin) is a natural compound that belongs to the class of 3-O-methylated metabolites of quercetin. Isorhamnetin demonstrates both preventive and therapeutic effects on cardiovascular and cerebrovascular diseases, including anti-atherosclerotic properties, protection of endothelial cells, anti-myocardial ischemia effects, anti-hypotension actions, anti-hypoglycemic effects, and anti-thrombotic properties, among others.13 The mechanisms underlying these beneficial effects are associated with its antioxidant, anti-inflammatory, and anti-mitochondrial-dependent effects on cell apoptosis.10-
Previous studies have indicated that NFE2L2 plays a crucial role in enhancing the body’s defense against ferroptosis. NFE2L2 regulates iron balance and the ferroptosis process through HERC2, VAMP8, and NCOA4. Elevated levels of NFE2L2 amplify its effects.43 Consequently, we conducted molecular docking studies to investigate the binding capabilities of isorhamnetin to NFE2L2 and other transcription factors (VEGFR, ATM, and ZNF24) associated with VEGF signaling. Our findings revealed that isorhamnetin exhibited strong affinities for these crucial regulators of PAH. These results suggest that isorhamnetin could be a promising natural compound in the fight against PAH.
Conclusion
Based on the results of the current analysis, we found that PAH is characterized by a series of abnormalities in downstream molecular signaling pathways, including DNA damage, immune dysregulation, VEGF signaling deficiency, and the ferroptosis process. These factors may be the core pathophysiological mechanisms of PAH. Ferroptosis-related genes, such as NFE2L2 and TF (including ATM and ZNF24) associated with VEGF signaling, serve as potential candidate therapeutic targets that contribute to the mechanisms mentioned above. Isorhamnetin is identified as a candidate compound for the treatment of PAH.
Footnotes
References
- Humbert M, Kovacs G, Hoeper MM. 2022 ESC/ERS Guidelines for the diagnosis and treatment of pulmonary hypertension. Eur Respir J. 2023;61(1):1-144.
- Omura J, Habbout K, Shimauchi T. Identification of long noncoding RNA H19 as a new biomarker and therapeutic target in right ventricular failure in pulmonary arterial hypertension. Circulation. 2020;142(15):1464-1484.
- Sumneang N, Siri-Angkul N, Kumfu S, Chattipakorn SC, Chattipakorn N. The effects of iron overload on mitochondrial function, mitochondrial dynamics, and ferroptosis in cardiomyocytes. Arch Biochem Biophys. 2020;680():108241-.
- Zou HX, Qiu BQ, Lai SQ. Iron metabolism and idiopathic pulmonary arterial hypertension: new insights from bioinformatic analysis. BioMed Res Int. 2021;2021():5669412-.
- Zhang F, Liu H. Identification of ferroptosis-associated genes exhibiting altered expression in pulmonary arterial hypertension. Math Biosci Eng. 2021;18(6):7619-7630.
- Miyamoto HD, Ikeda M, Ide T. Iron Overload via heme Degradation in the endoplasmic reticulum Triggers Ferroptosis in myocardial ischemia-reperfusion Injury. JACC Basic Transl Sci. 2022;7(8):800-819.
- Günes Günsel G, Conlon TM, Jeridi A. The arginine methyltransferase PRMT7 promotes extravasation of monocytes resulting in tissue injury in COPD. Nat Commun. 2022;13(1):1303-.
- Xie SS, Deng Y, Guo SL. Endothelial cell ferroptosis mediates monocrotaline-induced pulmonary hypertension in rats by modulating NLRP3 inflammasome activation. Sci Rep. 2022;12(1):3056-.
- Cristina Marcarini J, Ferreira Tsuboy MS, Cabral Luiz R, Regina Ribeiro L, Beatriz Hoffmann-Campo C, Ségio Mantovani M. Investigation of cytotoxic, apoptosis-inducing, genotoxic and protective effects of the flavonoid rutin in HTC hepatic cells. Exp Toxicol Pathol. 2011;63(5):459-465.
- Dong GZ, Lee JH, Ki SH. AMPK activation by isorhamnetin protects hepatocytes against oxidative stress and mitochondrial dysfunction. Eur J Pharmacol. 2014;740():634-640.
- Chi G, Zhong W, Liu Y. Isorhamnetin protects mice from lipopolysaccharide-induced acute lung injury via the inhibition of inflammatory responses. Inflamm Res. 2016;65(1):33-41.
- Sun M, Deng R, Wang Y. Sphingosine kinase 1/sphingosine 1-phosphate/sphingosine 1-phosphate receptor 1 pathway: A novel target of geniposide to inhibit angiogenesis. Life Sci. 2020;256():117988-.
- Gong G, Guan YY, Zhang ZL. Isorhamnetin: a review of pharmacological effects. Biomed Pharmacother. 2020;128():110301-.
- Ren X, Han L, Li Y. Isorhamnetin attenuates TNF-α-induced inflammation, proliferation, and migration in human bronchial epithelial cells via MAPK and NF-κB pathways. Anat Rec (Hoboken). 2021;304(4):901-913.
- Li S, Cheng CS, Zhang C. Edible and herbal plants for the prevention and management of COVID-19. Front Pharmacol. 2021;12():656103-.
- Mura M, Cecchini MJ, Joseph M, Granton JT. Osteopontin lung gene expression is a marker of disease severity in pulmonary arterial hypertension. Respirology. 2019;24(11):1104-1110.
- Yu G, Wang LG, Han Y, He QY. clusterProfiler: an R package for comparing biological themes among gene clusters. Omics. 2012;16(5):284-287.
- Liu Z, Zhao Q, Zuo ZX. Systematic analysis of the aberrances and functional implications of ferroptosis in cancer. iScience. 2020;23(7):101302-.
- Zhou Y, Zhou B, Pache L. Metascape provides a biologist-oriented resource for the analysis of systems-level datasets. Nat Commun. 2019;10(1):1523-.
- Han H, Cho JW, Lee S. TRRUST v2: an expanded reference database of human and mouse transcriptional regulatory interactions. Nucleic Acids Res. 2018;46(D1):D380-D386.
- Miao YR, Zhang Q, Lei Q. ImmuCellAI: A unique method for comprehensive T-cell subsets abundance prediction and its application in cancer immunotherapy. Adv Sci (Weinh). 2020;7(7):1902880-.
- Miao YR, Xia M, Luo M, Luo T, Yang M, Guo AY. ImmuCellAI-mouse: a tool for comprehensive prediction of mouse immune cell abundance and immune microenvironment depiction. Bioinformatics. 2022;38(3):785-791.
- Zeng D, Li M, Zhou R. Tumor microenvironment characterization in gastric cancer identifies prognostic and immunotherapeutically relevant gene signatures. Cancer Immunol Res. 2019;7(5):737-750.
- Wang J, Sun J, Liu LN. Siglec-15 as an immune suppressor and potential target for normalization cancer immunotherapy. Nat Med. 2019;25(4):656-666.
- Deng S, Zhang Y, Wang H. ITPRIPL1 binds CD3ε to impede T cell activation and enable tumor immune evasion. Cell. 2024;187(9):2305-2323.e33.
- Davis AP, Wiegers TC, Johnson RJ, Sciaky D, Wiegers J, Mattingly CJ. Comparative Toxicogenomics Database (CTD): update 2023. Nucleic Acids Res. 2023;51(D1):D1257-D1262.
- Wiesmann C, Fuh G, Christinger HW, Eigenbrot C, Wells JA, de Vos AM. Crystal structure at 1.7 A resolution of VEGF in complex with domain 2 of the Flt-1 receptor. Cell. 1997;91(5):695-704.
- Sengoku T, Shiina M, Suzuki K. Structural basis of transcription regulation by CNC family transcription factor, Nrf2. Nucleic Acids Res. 2022;50(21):12543-12557.
- Eberhardt J, Santos-Martins D, Tillack AF, Forli S, AutoDock V. AutoDock Vina 1.2.0: New docking methods, expanded force field, and python bindings. J Chem Inf Model. 2021;61(8):3891-3898.
- Warren C, Pavletich NP. Structure of the human ATM kinase and mechanism of Nbs1 binding. eLife. 2022;11():-.
- Ravindranath PA, Forli S, Goodsell DS, Olson AJ, Sanner MF. AutoDockFR: advances in protein-ligand docking with explicitly specified Binding Site flexibility. PLOS Comput Biol. 2015;11(12):e1004586-.
- Fu L, Shi S, Yi J. ADMETlab 3.0: an updated comprehensive online ADMET prediction platform enhanced with broader coverage, improved performance, API functionality and decision support. Nucleic Acids Res. 2024;52(W1):W422-W431.
- Li B, Rui J, Ding X, Yang X. Exploring the multicomponent synergy mechanism of Banxia Xiexin Decoction on irritable bowel syndrome by a systems pharmacology strategy. J Ethnopharmacol. 2019;233():158-168.
- Ma C, Wang X, Zhang L. Super enhancer-associated circular RNA-CircKrt4 regulates hypoxic pulmonary artery endothelial cell dysfunction in mice. Arterioscler Thromb Vasc Biol. 2023;43(7):1179-1198.
- Chakraborty A, Nathan A, Orcholski M. Wnt7a deficit is associated with dysfunctional angiogenesis in pulmonary arterial hypertension. Eur Respir J. 2023;61(6):1-47.
- Lan M, Wu S, Fernandes TM. Iron deficiency and pulmonary arterial hypertension. Nutr Clin Pract. 2022;37(5):1059-1073.
- Thenappan T, Ormiston ML, Ryan JJ, Archer SL. Pulmonary arterial hypertension: pathogenesis and clinical management. BMJ. 2018;360():j5492-.
- Zhang DD. Mechanistic studies of the Nrf2-Keap1 signaling pathway. Drug Metab Rev. 2006;38(4):769-789.
- Mitsuishi Y, Taguchi K, Kawatani Y. Nrf2 redirects glucose and glutamine into anabolic pathways in metabolic reprogramming. Cancer Cell. 2012;22(1):66-79.
- Pajares M, Jiménez-Moreno N, García-Yagüe ÁJ. Transcription factor NFE2L2/NRF2 is a regulator of macroautophagy genes. Autophagy. 2016;12(10):1902-1916.
- Liu P, Dodson M, Li H. Non-canonical NRF2 activation promotes a pro-diabetic shift in hepatic glucose metabolism. Mol Metab. 2021;51():101243-.
- Anandhan A, Dodson M, Shakya A. NRF2 controls iron homeostasis and ferroptosis through HERC2 and VAMP8. Sci Adv. 2023;9(5):eade9585-.
- Yang JH, Shin BY, Han JY. Isorhamnetin protects against oxidative stress by activating Nrf2 and inducing the expression of its target genes. Toxicol Appl Pharmacol. 2014;274(2):293-301.